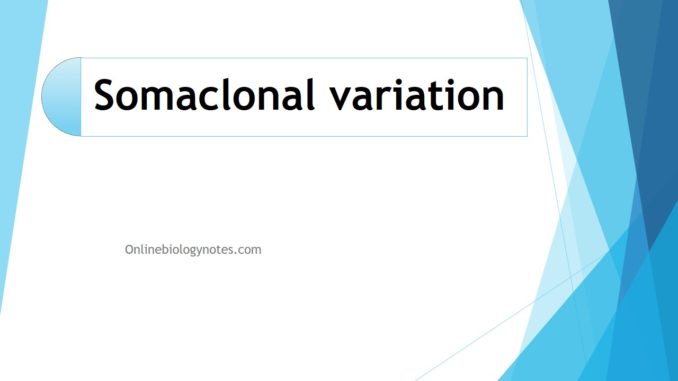
Somaclonal variation
- The term somaclonal variation by Larkin and Scowcroft (1981) was given for the variability generated by the use of a tissue culture cycle.
- Somaclonal variation is defined as genetic variation observed among progeny plants obtained after somatic tissue culture in vitro.
- Theoretically all progeny plants regenerated from somatic cells should be identical clones. However, variations might occur in number of progeny which are known as somaclones and they are genetically variable from their explant.
- The initiating explant for a tissue culture cycle may come virtually from any plant organ or cell type including embryos, microspores, roots, leaves and protoplasts. So, all somatic tissue culture can result in somaclonal variation.
- Somaclonal variation is a phenotypic changes as a result of chromosomal rearrangement during tissue culture.
- Nomenclature of somaclonal variation:
- Though different letters and symbols have been used, two symbols are generally used.
- Chaleff (1981) has labelled the plants regenerated from tissue culture as R or R0 plants and the self-fertilized progeny of R0 plants as R1.
- Subsequent generations produced by self-fertilization are termed R2, R3, R4, etc. Larkin and Scowcroft (1981) have referred regenerated plants as SC1 (=R0) and subsequent self-fertilized generations as SC2, SC3, SC4, etc
Basis of somaclonal variation:
- Following are some of the basis of chromosomal rearrangement which results in somaclonal variation
- Karyotype changes
- Changes in chromosome structure
- Single gene mutations
- Cytoplasmic genetic changes
- Mitotic crossing over
- Gene amplification and nuclear changes
- Transposable elements
- DNA methylation
1. Karyotype changes:
- Variant plants with altered chromosome number have been reported by several workers.
- Polyploidy is the most frequently observed chromosome abnormality e.g. aneuploidy in oats, potato, barley.
- The change in chromosome number in a variant plant is commonly associated with reduced fertility and with altered genetic ratios in the progeny of self-fertilized plants.
2. Changes in chromosome structure:
- In contrast to gross changes in chromosome number, more cryptic chromosome rearrangements may be responsible for genetic variation.
- Translocations have been reported in potato, ryegrass, oats, etc. Published work also suggests that chromosome deletions, duplications, inversions and other minor reciprocal and non-reciprocal rearrangements occur among regenerated plants e.g. ryegrass, barley.
- Chromosome irregularities such as breaks, acentric and centric fragments, ring chromosomes and micronuclei were observed in garlic somaclones.
- Cytological abnormalities such as multilobed nuclei, multinucleate cells, abnormal anaphase and mixoploidy were observed in self-fertilized, SC3 generation of barley.
3. Single gene mutations:
- Single gene mutations have been detected by several workers (Edallo et al., 1981; Oono, 1978).
- Recessive single gene mutations are suspected if the variant does not appear in Ro or SC1 generation but the self-fertilized R1 or SC2 progeny segregate in 3:1 Mendelian ratio for the trait of interest. This type of analysis has been reported for maize, Nicotiana sylvestris, rice, wheat, etc.
4. Cytoplasmic genetic changes:
- The most detailed work on this has been done on maize.
- Many studies have implicated mitochondria as the determinants of male sterility and Drechslera maydis T toxin sensitivity in maize.
- The original lines used had all the Texas male sterile cytoplasm, which results in plants being normally highly susceptible to this disease.
- However, when selection pressure was applied by subculturing callus cultures in the presence of toxin, some regenerants were found to be not only resistant to the toxin but also fertile.
- When restriction analysis of the mitochondrial DNA (mtDNA) was evaluated it was evident that significant changes had occurred in the mtDNA of plants derived from cell cultures.
- Molecular analyses based on restriction endonuclease digest patterns of mitochondrial and chloroplast genomes of regenerated potato plants derived from protoplasts have revealed significant change in mitochondrial genomes but not in chloroplast genomes.
- It was suggested that the variation results from substantial DNA sequence rearrangements (e.g. deletion, addition and intramolecular recombination) and cannot be explained by simple point mutations.
- However, point mutations can also occur in the genomes of organelles of regenerated plants.
- Variations in chloroplast DNA have been detected in tomato and wheat somaclones.
5. Mitotic crossing over:
- Mitotic crossing over (MCO) could also account for some of the variation detected in regenerated plants.
- This could include both symmetric and asymmetric recombination. MCO may account for the recovery of homozygous recessive single gene mutations in some regenerated plants.
- Somatic cell sister chromatid exchange, if it is asymmetric can also lead to deletion and duplication of genetic material and hence variation.
6. Gene amplification and nuclear changes:
- Studies have shown that nuclear genes are affected by tissue culture stress.
- It has been seen that heritable quantitative and qualitative changes can be observed in the nuclear DNA content of doubled haploid Nicotiana sylvestris obtained from pollen cultured plants.
- These plants contain generally increased amounts of total DNA and an increasing proportion of highly repeated sequences.
- Both AT and GC rich fractions are amplified in tissue culture derived plants. Durante et al. (1983) showed similar amplification of AT and GC rich fractions in DNA from Nicotiana glauca pith explants within hours of culture.
- These studies suggest the presence of a differential replication process during the early stages of dedifferentiation.
- It has been seen that plant cells like those of other eukaryotes can increase or decrease the quantity of a specific gene product by differential gene amplification and diminution.
- For instance, ribosomal RNA gene amplification and diminution are now known to be widespread in wheat, rye, and tobacco, and in flax, ribosomal DNA is known to alter directly in response to environmental and cultural pressures.
- Reduction in ribosomal RNA genes (rDNA) has been found in potato plants regenerated from protoplasts.
- Additionally, both structural rearrangement within rDNA and methylation of nucleotide sequences of rDNA have been observed.
7. Transposable elements:
- Several authors have speculated that transposable elements may also be responsible for somaclonal variation.
- Heterozygous light green (Su/su) somaclones with a high frequency of coloured spots on the leaf surface have been detected for a clone of N. tabacum and for a N. tabacum + N. sylvestris somatic hybrid. The somatic hybrid has an unstable pattern of inheritance that would be consistent with an unstable gene.
- A causal relationship between genetic instability possibly related to tissue culture induced transposition and somaclonal variation was speculated.
- Recently, genomic changes in a maize line and mutations in tobacco line due to activation of transposable elements under in vitro tissue culture conditions have been demonstrated.
- It has also been shown that several types and copies of non-active transposable elements, including retro- transposable elements, are present in the genome of potato
- Thus, it is likely that a few active transposable elements may also be present in potato and could be responsible for generating some degree of somaclonal variation in regenerated potato plants.
8. DNA methylation:
- DNA methylation plays an important role in the regulation of gene expression and its implication in somaclonal variation.
- Many genes show a pattern in which the state of methylation is constant at most sites but varies at others.
- A majority of sites are methylated in tissues in which the gene is not expressed but non-methylated in tissues where the gene is active. Thus, an active gene may be described as unmethylated.
- A reduction in the level of methylation is part of some structural change needed to permit transcription to proceed.
- Important changes in the methylation level of genomic DNA in course of dedifferentiation and somatic embryogenesis have been reported for higher plants.
- Wherever the gene expression and its regulation is playing a role, DNA methylation becomes an important factor for getting the somaclonal variants.
- Unstability of gene may arise through the methylation pattern of that particular gene and thus a causal relationship between genetic instability possibly related to tissue culture-induced transposition, and somaclonal variation.
- In higher plants many controlling factors act together to achieve the desired gene regulation.
- One of these levels of control is provided by adding a small “tag” called a methyl group onto “C” one of the bases that make up the DNA code.
- The methyl group tagged C’s can be written as mC.
- Simpler organisms, such as many types of bacteria and the single celled yeast, usually do not use methyl group tagged C’s in regulating their genes, some bacteria but not all use methyl group tagged A’s “mA” for this purpose.
- However, most bacteria have specific patterns of mC and mA for this purpose i.e. for signalling in their DNA called methylated DNA.
- Thus the process in which the methyl group is added to certain bases as in cytosine (C) and adenine (A) by the help of enzyme DNA methylase at the C5 atom of cytosine and N6 position of adenine is called methylation.
- When only one strand of DNA is methylated it is called hemi-methylated DNA if it is in both strands of DNA this is called fully methylated DNA.
- In hemi-methylated condition gene is able to express while in fully methylated state gene cannot be expressed.
- In general rule, methylation prevents the gene expression but the de-methylation respond to the expression of a gene.
- Hence in terms of somaclonal variation (i.e. variation occurs in the in vitro culture of plants) the DNA methylation by regulating the gene expression and sometimes by inducing the mutations may cause variation in the culture.
- In Arabidopsis thaliana, a new variant has been developed from the normal one this is a tiny dwarf plant, shriveled, a mere shadow of its genetically identical neighbour
- This dwarf plant is named as “bal”, because of its shape.
- It constantly perceives a pathogen attack even though it has the exact same DNA sequence of parental plant.
- It was found that the difference between the two plants is not due to changes in the DNA sequence but actually the ‘bal dwarf’ is caused by increased activation of a single gene.
- This is something looks like a mutation and behaves like a mutation but it is actually caused by the packaging of DNA (caused basically by DNA methylation) rather than change in the DNA sequence.
- The gene affected in ‘bal variant’ is involved in the disease resistance and is called an R-gene.
- The R-gene is more active in ‘bal plant’ and as a result the plant defense system becomes hyperactivated, constantly fighting off disease even when no pathogens are present to pose a threat.
- The yielded dwarf plant is more resistant to bacterial infection.
- The precise molecular change leading to the increased R-gene activation is most probably the changes in DNA methylation, chemical modification of cytosine.
Applications of Somaclonal Variation:
i. Production of Novel variants:
- An implication of somaclonal variation in breeding is that novel variants can arise and these can be agronomically used.
- A number of breeding lines have been developed by somaclonal variation.
- Example: An enhanced scented Geranium variety named ‘Velvet Rose’ has been generated. An example of heritable somaclonal variation is the development of pure thornless blackberries Lincoln Logan (Rubus), Hasuyume, a protoplast derived rice cultivar, and somaclone T-42 has been generated.
- In India two varieties namely Pusa Jai Kisan in mustard Brassica and CIMAP/Bio13 in Citronella have been released for cultivation.
- An improved variety of rice ‘DAMA’ has been released through pollen haploid somaclone method which combined microspore culture with somatic tissue culture (see Heszky and Simon-Kiss, 1992).
- Somaclonally derived mutants in tomato with altered color, taste, texture and shelf life are being marketed in USA by Fresh World farms
ii. Production of disease resistance variety:
- The greatest contribution of somaclonal variation towards plant improvement is in the development of disease resistant genotypes in various crop species.
- Resistance was first reported in sugarcane for eye spot disease (Helminthosporium sacchari), downy mildew (Sclerospora sacchari) and Fiji virus disease by regenerating plants from the callus of susceptible clones and screening the somaclones.
- Following table lists few successful examples of somaclonal variants obtained without in vitro selection at the plant level with an increased disease resistance:
Food crops | Pathogen |
Barley | Rhynchosporium secalis |
Maize | Helminthosporium maydis |
Rice | Helminthosporium oryzae |
Rape | Phoma lingam, Alternaria brassicicola |
Sugar–cane | Fijivirus, Sclerospora saccharii, Helminthosporium sacchari |
iii. Production of abiotic stress resistance variety:
- Somaclonal variation has resulted in several interesting biochemical mutants, which are being successfully used in plant metabolic pathway studies, i.e. amino acid and secondary metabolic pathways.
- Investigations have shown that the level of free amino acids, especially proline, increases during cold hardening.
- In vitro selection has also been used to obtain plants with increased acid soil, salt, aluminium and herbicide resistance.
iv. Production of Cold tolerance:
- Lazar et al. (1988) developed somaclonal variants for freezing tolerance in Norstar winter wheat.
- A significant positive correlation between proline level and frost tolerance has been found in a broad spectrum of genotypes.
- In vitro selection and regeneration of hydroxyproline resistant lines of winter wheat with increased frost tolerance and increased proline content has been reported (Dorffling et al., 1997).
- The results showed strong correlation of increased frost tolerance with increased proline content.
v. Production of Salt tolerance:
- Plant tissue culture techniques have been successfully used to obtain salt tolerant cell lines or variants in several plant species, viz. tobacco, alfalfa, rice, maize, Brassica juncea, Solanum nigrum, sorghum, etc.
- In most cases, the development of cellular salt tolerance has been a barrier for successful plant regeneration, or if plants have been obtained they did not inherit the salt tolerance.
- Only in a few cases it was possible to regenerate salt tolerant plants. Mandal et al. (1999) developed a salt tolerant somaclone BTS24 from indigenous rice cultivar Pokkali.
- This somaclone yielded 36.3 q/ha under salt stress conditions as compared to 44 q/ha under normal soil.
- Some of the reports for successful production of healthy, fertile and genetically stable salt tolerant regenerants from various explants.
vi. Production of Aluminium tolerance:
- Plant species or cultivars greatly differ in their resistance to aluminium stress.
- In recent years, considerable research has been focused on the understanding of physiological, genetic and molecular processes that lead to aluminium tolerance.
- Despite the problems encountered in adapting culture media for in vitro selection for aluminium resistance, cell lines have been isolated in several species, e.g. alfalafa, carrot, sorghum, tomato, tobacco.
- Aluminium tolerant somaclonal variants from cell cultures of carrot were selected by exposing the cells to excess ionic aluminium in the form of aluminium chloride (Arihara et al., 1991).
- Jan et al. (1997) elicited aluminium toxicity during in vitro selection in rice by making several modifications in the media viz. low pH, low phosphate and calcium concentrations, and unchelated iron and aluminium along with aluminium sulphate.
- After selection on aluminium toxic media, callus lines were maintained on aluminium toxic free media and 9 tolerant plants were obtained. Transmission of aluminium resistance character was identified till the fourth generation.
vii. Production of Drought tolerance:
- Wang et al. (1997) reported a sorghum somaclonal variant line (R111) resistant to drought stress.
- A novel hybrid was developed by crossing R111 with several sterile lines, suggesting that selection of somaclonal variants is an effective method for creating new sources of genetic variation.
- Drought tolerant rice lines were obtained by in vitro selection of seed induced callus on a media containing polyethylene glycol as a selective agent which simulated the effect of drought in tissue culture conditions.
viii. Production of Herbicide resistance:
- Through in vitro selection several cell lines resistant to herbicides have been isolated and a few have been regenerated into complete plants.
- Among the important achievements are tobacco, soybean, wheat, maize, etc. resistant to various herbicides such as glyphosate, sulfonylurea, imidazolinones, etc.
ix. Production of Insect resistance:
- Zemetra et al. (1993) used in vitro selection technique for generation of somaclonal variants for Russian wheat aphid (Diuraphis noxia) in wheat. Calli from susceptible wheat cultivar “Stephens’’ were exposed to an extract from aphid. Resistance to aphid was observed in both R2 and R3 generations.
x. Seed quality improvement:
- Recently, a variety Bio L 212 of lathyrus (Lathyrus sativa) has been identified for cultivation in central India which has been developed through somaclonal variation and has low ODAP (β-N-oxalyl -2-α, β diamino propionic acid), a neurotoxin (Mehta and Santha, 1996), indicating the potential of somaclonal variations for the development of varieties with improved seed quality.
xi. Introgression of Alien gene:
- The increase in genome rearrangement during tissue culture provides a new opportunity for alien gene introgression which can help widen the crop germplasm base, particularly by culturing immature embryos of wide crosses where crop and alien chromosomes cannot replicate through meiosis.
- Introgression of genes may be better achieved by imposing one or more cell culture cycles on interspecific hybrid material.
- The enhanced somatic genome exchange is likely to produce regenerants where part of the alien genome has been somatically recombined into the chromosomes of crop species.
Limitations of somaclonal variation:
- Uncontrollable and unpredictable nature of variation and most of the variations are of no apparent use.
- The variation is cultivar dependent.
- The variation obtained is not always stable and heritable. The changes occur at variable frequencies.
- Not all the changes obtained are novel. In majority of cases, improved variants have not been selected for breeding purposes.