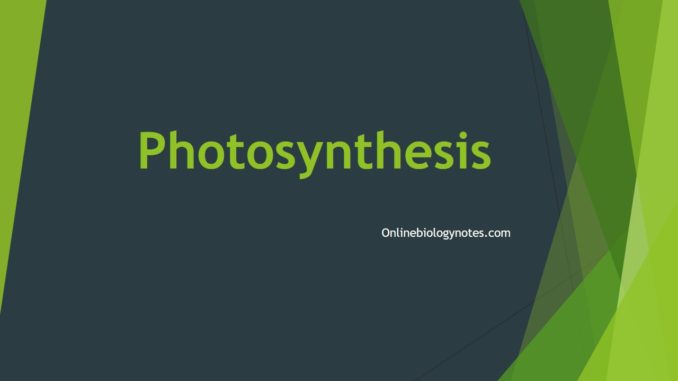
What is photosynthesis?
- The process of synthesis with the assistance of light is termed as photosynthesis.
- It is the mechanism by which sunlight is used by green plants to produce food from simple molecules like CO2 and H2O.
- Often called carbon assimilation, photosynthesis is expressed by the following equation;
- 6CO2 + 6H2O——— > C6 H12 O6 + 6O2
- The light energy is transformed into chemical energy during the photosynthesis process and is stored into the organic matter, which is normally carbohydrates, and O2 is a by-product of photosynthesis.
- The raw materials for this process are CO2 and H2O.
- CO2 from the air and H2O from the soil are absorbed.
- The following points make photosynthesis essential to humanity:
- It preserves the atmospheric equilibrium of O2.
- It provides food either directly as vegetables or indirectly as animal meat or milk which, in turn, is fed to plants.
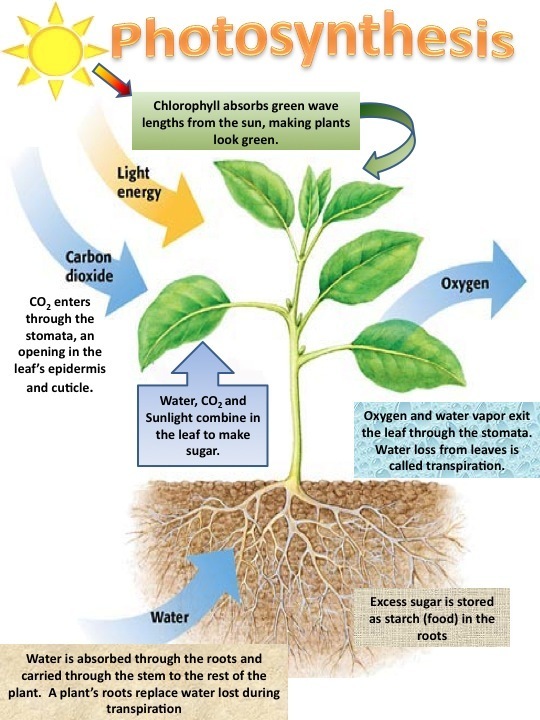
Where does photosynthesis takes place?
- The photosynthesis occurs in chloroplasts in case of plants and blue-green algae.
- When microscopic observation of a leaf section is done, chlorophyll is not uniformly distributed in the cell.
- However, it is confined to organelles termed as chloroplasts.
- In plants, chloroplasts lie mainly in the cells of mesophyll, a layer that includes several air spaces and a very high concentration of water vapor.
- The exchange of gases takes place between interior of the leaf and the outside through microscopic pores, termed as stomata.
- Each mesophyll cell consists of 20-100 chloroplasts.
- The chloroplast is enclosed by outer and inner membranes.
- The inner membrane encloses a fluid filled region termed as stroma.
- Stroma consists of most of the enzymes needed to produce carbohydrate molecules.
- The third system of membranes is suspended in the stroma.
- This system forms an interconnected set of flat, disc like sacs called thylakoids.
- A fluid-filled internal space, the thylakoid lumen, is enclosed by the thylakoid membrane.
- Thylakoid sacs are arranged in stacks called grana (sing., granum) in some regions of the chloroplast.
- Each granum is identical to a coin stack, with a thylakoid being each “coin.”
- Some thylakoid membranes extend from one granum to another.
- Thylakoid membranes are involved in ATP synthesis.
Photosynthetic pigments:
- There are major three photosynthetic pigments. They are:
- Chlorophylls
- Carotenoid
- Phycobillins
i. Chlorophylls:
- Chlorophylls are present in thylakoid membranes.
- Thylakoid membranes consist of several kinds of pigments that absorb visible light.
- Various pigments absorb light from various wavelengths.
- Chlorophyll is the dominant photosynthesis pigment and it absorbs light mainly in the visible spectrum’s blue and red areas.
- Green light is not appreciably absorbed by chlorophyll.
- Some of the green light that strikes the plant is either scattered or reflected and hence the plants typically appear to be green in colour.
- There are two principal parts of a chlorophyll molecule, a complex ring and a long side chain.
- A porphyrin ring is ring structure that comprises of smaller rings made of carbon and nitrogen atoms.
- The porphyrin ring absorbs light energy.
- The chlorophyll porphyrin ring is remarkably similar to the red pigment hemoglobin component of the heme in red blood cells.
- However, unlike heme, that holds an atom of iron in the center of the ring, chlorophyll consists of an atom of magnesium in that position.
- The chlorophyll molecule also contains a long, hydrocarbon side chain that makes the molecule extremely nonpolar and ties up the chlorophyll in the membrane
- All chlorophyll molecules in the thylakoid membrane are associated with specific chlorophyll-binding proteins
- Each thylakoid membrane is filled with accurately oriented chlorophyll molecules and chlorophyll-binding proteins that facilitate the transfer of energy from one molecule to another.
- There are many kinds of chlorophyll.
- The chlorophyll ‘a’ is the most essential pigment that starts the light-dependent reactions of photosynthesis.
- Chlorophyll ‘b’ is the accessory pigment which also takes part in photosynthesis.
- It differs from chlorophyll ‘a’ only in a functional group on the porphyrin ring.
- The methyl group (-CH3) in chlorophyll ‘a’ is replaced in chlorophyll ‘b’ by a terminal carbonyl group (-CHO).
- This difference shifts the wavelengths of light absorbed and reflected by chlorophyll ‘b’.
- It makes it to appear yellow-green, whereas chlorophyll ‘a’ appears bright green.
ii. Carotenoids:
- Chloroplasts have other accessory photosynthetic pigments, such as carotenoids, that are of two types:
- Carotenes (orange)
- Xanthophyll (yellow)
- Carotenoids absorb different wavelengths of light than chlorophyll.
- Thus it expands the spectrum of light that provides energy for photosynthesis.
- Chlorophyll may be excited by light directly by energy passed to it from the light source or indirectly by energy passed to it from accessory pigments that have become excited by light.
- When a carotenoid molecule is excited, its energy can be transferred to chlorophyll a.
- In addition, carotenoids are antioxidants that inactivate highly reactive forms of oxygen generated in the chloroplasts hence termed as shield pigment.
iii. Phycobillins:
- It is water soluble pigment.
- It consists of 4 pyrrol rings that lacks Mg and phytol tail.
- It is responsible for maximum absorption in green parts of the spectrum.
- It is distributed in case of red and blue algae.
- Phycoerythrin are distributed in red algae and phycocyanin are distributed in red as well as blue algae.
What are the stages of photosynthesis?
- Photosynthesis consists of two phases.
- Light dependent reaction (grana reaction or Hill reaction or Photochemical reaction or Primary photochemical reaction)
- Dark reactions (light independent reaction or stroma reaction or Blackman’s reaction or carbon-fixation reactions)
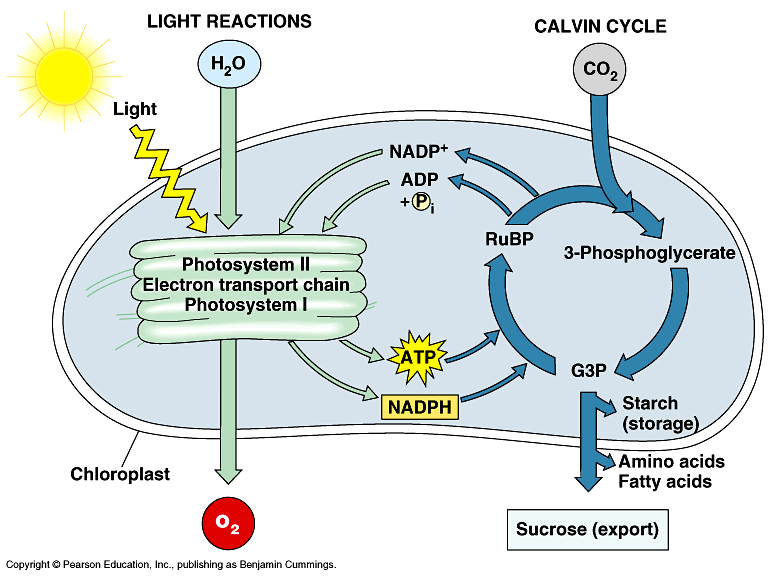
Stage I: Light dependent reaction/ Hill reaction:
- Light reaction occurs in grana of chloroplast and it requires the light for it to take place.
- Green plants uses light belonging to visible spectrum (380nm-760nm).
- In light reaction, the light energy is converted into chemical energy in the forms of ATP, NADPH+H+, and oxygen.
- According to Robert Hill and Bendall, light reaction comprises of two steps i.e. photolysis and photophosphorylation.
- Light reaction has four successive steps:
- Absorption
- Activation
- Photolysis
- Photophosphorylation
Photolysis:
- The light reaction initiates when the chlorophyll absorbs light. It causes one of its electrons to move to a higher energy state.
- The excited electron is transferred to an acceptor molecule and is replaced by an electron from water (H2O).
- It causes the decomposition of water into H+ and OH–, hence termed as photolysis.
Photo-phosphorylation:
- Chlorophylls a and b and accessory pigment molecules are arranged with pigment-binding proteins in the thylakoid membrane into units called antenna complexes.
- The pigments and related proteins are organized as highly ordered groups comprising approximately 250 molecules of chlorophyll associated with particular enzymes and other proteins.
- Each antenna complex absorbs light energy and transfers it to its reaction system.
- This reaction system consists of chlorophyll molecules and proteins, including electron transfer components directly involved in photosynthesis.
- The light energy is converted into chemical energy in the reaction centers by a series of electron transfer reactions.
- Photosynthesis requires two types of photosynthetic units, referred to as photosystem I and photosystem II.
- Their reaction centers can be differentiated as they are related to proteins in a way that induces a slight difference in their absorption spectra.
- Normally, chlorophyll a has a strong absorption peak at about 660 nm.
- In contrast, the reaction center of photosystem I consists of a pair of chlorophyll a molecules with an absorption peak at 700 nm and is referred to as P700.
- The reaction center of photosystem II is made up of a pair of chlorophyll a molecules with an absorption peak of about 680 nm and is referred to as P680
- Photophosphorylation is further divided into two types:
- i. Non-cyclic photophosphorylation:
- Photosystem II becomes activated when a pigment molecule in an antenna complex absorbs a photon of light energy.
- The energy is transferred to the reaction center, where it causes an electron in a molecule of P680 to move to a higher energy level.
- This energized electron is accepted by a primary electron acceptor (a highly modified chlorophyll molecule known as pheophytin).
- Then it passes along an electron transport chain until it is donated to P700 in photosystem I.
- A pigment molecule in an antenna complex associated with photosystem I absorbs a photon of light.
- The absorbed energy is transferred from one pigment molecule to another until it reaches the reaction center, where it excites an electron in a molecule of P700.
- This energized electron is transferred to a primary electron acceptor, which is the first of several electron acceptors in a series.
- The energized electron is passed along an electron transport chain from one electron acceptor to another, until it is passed to ferredoxin, an iron-containing protein.
- Ferredoxin transfers the electron to NADP+ in the presence of the enzyme ferredoxin–NADP+ reductase.
- Although single electrons pass down the electron transport chain, two are needed to reduce NADP+. When NADP+ accepts 2 electrons, they unite with a proton (H+); thus, the reduced form of NADP+ is NADPH, which is released into the stroma.
- P700 becomes positively charged when it gives up an electron to the primary electron acceptor.
- The missing electron is replaced by one donated by photosystem II.
- Noncyclic electron transport is a continuous linear process
- In the availability of light, there is a continuous, one- way flow of electrons from the ultimate electron source, H2O, to the terminal electron acceptor, NADP+.
- Water goes through enzymatically catalyzed photolysis to replace energized electrons donated to the electron transport chain by molecules of P680 in photosystem II.
- These electrons travel down the electron transport chain that connects photosystem II with photosystem I.
- Thus, they provide a continuous supply of replacements for energized electrons that have been given up by P700.
- As electrons are transferred along the electron transport chain that connects photosystem II with photosystem I, they lose energy.
- Some of the energy released is used to pump protons across the thylakoid membrane, from the stroma to the thylakoid lumen, producing a proton gradient.
- The energy of this proton gradient is utilized to produce ATP from ADP by chemiosmosis.
- ATP and NADPH, the products of the light-dependent reactions, are released into the stroma.
- Both are required by the carbon fixation reactions.
- ii. Cyclic photophosphorylation:
- Cyclic electron transport is the simplest light-dependent reaction.
- Only Photosystem I is involved in this reaction.
- The term cyclic is given for the pathway as the energized electrons that initiate from the P700 at the reaction finally return to P700.
- In the presence of light, electrons flow continuously through an electron transport chain within the thylakoid membrane.
- As the electrons pass from one acceptor to the other, the electrons lose energy.
- Some of the energy is used to pump protons across the thylakoid membrane.
- ATP synthase, an enzyme present in the thylakoid membrane uses the energy of the proton gradient to manufacture ATP.
- In the cyclic photophosphorylation, NADPH is not produced, H2O is not split, and oxygen is not generated.
- Hence, it cannot serve as basis for photosynthesis as NADPH is required to reduce CO2 to carbohydrate.
- The importance of cyclic electron transport to photosynthesis in plants is unclear.
- Cyclic electron transport may take place in plant cells when there is too little NADP+ to accept electrons from ferredoxin.
- There is a proof that cyclic electron flow may aid to maintain the optimal ratio of ATP to NADPH required for carbon fixation as well as provide extra ATP to power other ATP-requiring processes in chloroplasts.
- The process is termed as photophosphorylation as the synthesis of ATP (i.e. the phosphorylation of ADP) is coupled to the transport of electrons that have been energized by photons of light.
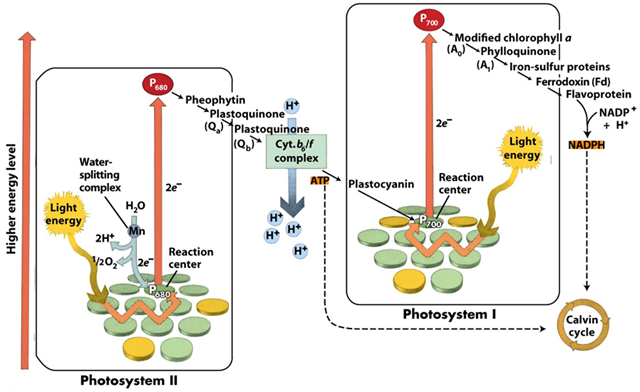
Stage II: Dark reaction/Carbon fixation reactions:
- In carbon fixation, the energy of ATP and NADPH is used in the formation of organic molecules from CO2 in carbon fixation.
- The carbon fixation reactions may be summarized as follows:
- 12NADPH + 18ATP + 6CO2 —> C6H12O6 + 12NADP+ +18ADP +18Pi+ 6H2O
- Carbon fixation occurs in the stroma of chloroplast and is again classified into two types:
- Calvin cycle/C3 cycle
- Hatch and Slack pathway or C4 cycle
i. Calvin cycle/C3 cycle:
- It comprises a series of 13 reactions.
- These reactions of the Calvin cycle are divided into three phases:
- Uptake of CO2
- Carbon reduction
- RuBP regeneration
- All 13 enzymes that catalyze steps in the Calvin cycle are located in the stroma of the chloroplast.
- These enzymes catalyze reversible reactions, degrading carbohydrate molecules in cellular respiration and synthesizing carbohydrate molecules in photosynthesis.
- Uptake of CO2:
- The first phase of the Calvin cycle consists of a single reaction.
- In this reaction, a molecule of CO2 reacts with a phosphorylated 5-carbon
compound, ribulose bisphosphate (RuBP). - This reaction is catalyzed by the enzyme ribulose bisphosphate carboxylase/oxygenase, also termed as rubisco.
- The chloroplast contains abundant rubisco enzyme than any other protein.
- The product of this reaction is an unstable 6-carbon intermediate, which instantly breaks down into 2 molecules of phosphoglycerate (PGA) with 3 carbons each.
- The carbon that was initially the part of a CO2 molecule is now part of a carbon skeleton; i.e. the carbon has been “fixed.”
- The Calvin cycle is also known as the C3 pathway asthe product of the initial carbon fixation reaction is a 3-carbon compound.
- Plants that initially fix carbon in this way are called C3 plants.
- Carbon reduction:
- The second phase of the Calvin cycle involves two steps in which the energy and reducing power from ATP and NADPH (both produced in the light-dependent reactions) are used to convert the PGA molecules to glyceraldehyde-3-phosphate (G3P).
- For every 6 carbons that enter the cycle as CO2, 6 carbons can leave the system as 2 molecules of G3P, to be used in carbohydrate synthesis.
- Each of these 3-carbon molecules of G3P is essentially half a hexose (6-carbon sugar) molecule.
- The reaction of 2 molecules of G3P is exergonic and leads to the formation of glucose or fructose.
- In some plants glucose and fructose are then joined to produce sucrose
- The plant cell also uses glucose to produce starch or cellulose.
- RuBP regeneration:
- Even if, two G3P molecules are eliminated from the cycle, 10 G3P molecules remains, this represents a total of 30 carbon atoms.
- Through a series of 10 reactions that make up the third phase of the Calvin cycle, these 30 carbons and their associated atoms become rearranged into 6 molecules of ribulose phosphate.
- Each of them becomes phosphorylated by ATP to produce RuBP, the 5-carbon compound with which the cycle started.
- These RuBP molecules begin the process of CO2 fixation and eventual G3P production once again.
- In summary, the inputs required for the carbon fixation reactions are 6 molecules of CO2, phosphates transferred from ATP, and electrons (as hydrogen) provided by NADPH (but ultimately derived from the photolysis of water).
- In the end, the 6 carbons from the CO2 are accounted for by the harvest of a hexose molecule.
- The remaining G3P molecules are used to synthesize the RuBP molecules with which more CO2 molecules may combine.
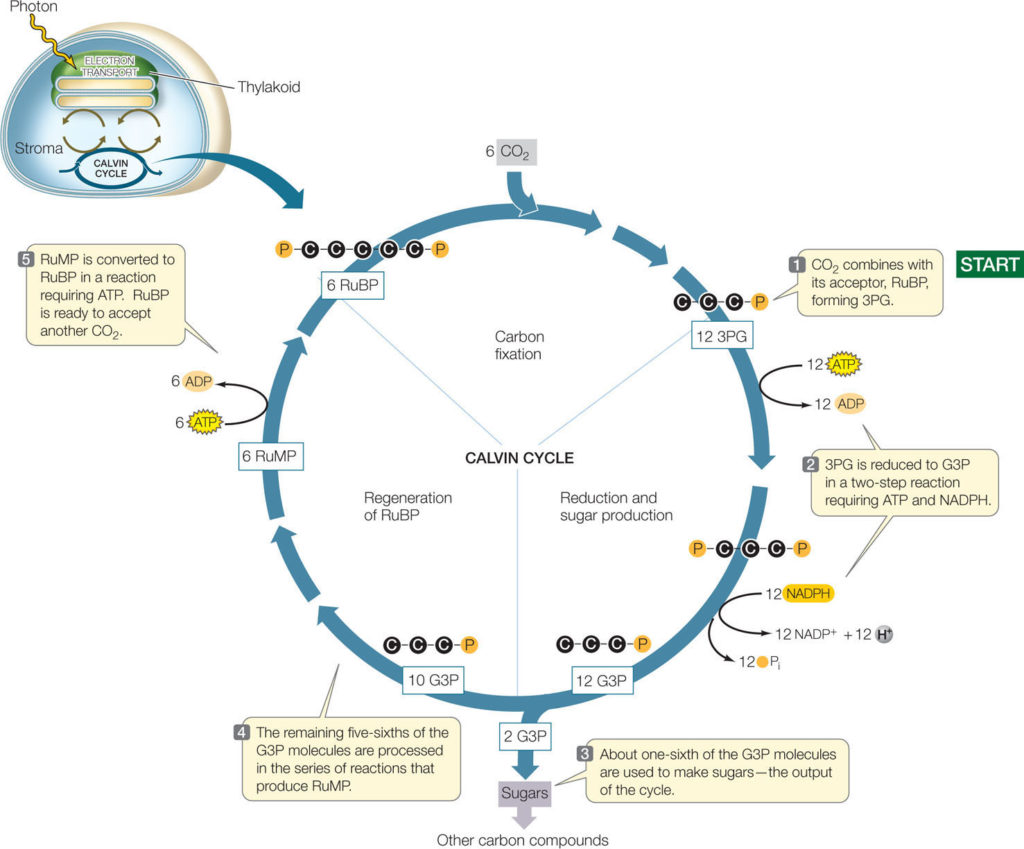
ii. Hatch and slack pathway/ C4 cycle:
- Several plant species found in hot, dry environments have adaptations that facilitate carbon fixation.
- C4 plants first fix CO2 into a 4-carbon compound, oxaloacetate.
- CAM plants initially fix carbon at night through the formation of oxaloacetate.
- These special pathways found in C4 and CAM plants precede the Calvin cycle (C3 pathway); they do not replace it.
- C4 pathway:
- The C4 pathway efficiently fixes CO2 at low concentrations.
- In this pathway, CO2 is fixed through the formation of oxaloacetate, occurs not only before the C3 pathway but also in different cells.
- Leaf anatomy is usually typical in C4 plants.
- The photosynthetic mesophyll cells are closely associated with prominent, chloroplast-containing bundle sheath cells, which tightly surrounds the veins of the leaf.
- The C4 pathway occurs in the mesophyll cells, whereas the Calvin cycle takes place within the bundle sheath cells.
- The major component of the C4 pathway is PEP carboxylase.
- It is a peculiar enzyme that has an extremely high affinity for CO2 i.e. binds it effectively even at unusually low concentrations.
- Carboxylation:
- PEP carboxylase catalyzes the reaction by which CO2 reacts with the 3-carbon compound phosphoenolpyruvate (PEP) and forms oxaloacetate.
- Breakdown:
- In a step that needs NADPH, oxaloacetate is converted to some other 4-carbon compound, malate and aspartate in presence of enzyme transaminase and malate dehydrogenase.
- Splitting:
- The malate and aspartate then passes to chloroplasts within bundle sheath cells.
- Here, a different enzyme catalyzes the decarboxylation of malate and yields pyruvate (which has 3 carbons) and CO2.
- NADPH is formed, replacing the one used earlier.
- Malate + NADP+ —-> pyruvate + CO2 + NADPH
- The CO2 released in the bundle sheath cell combines with ribulose bisphosphate in a reaction catalyzed by rubisco and undergoes the Calvin cycle in the usual manner.
- Phosphorylation:
- The pyruvate formed in the decarboxylation reaction returns to the mesophyll cell, where it reacts with ATP to regenerate phosphoenolpyruvate.
- As the C4 pathway captures CO2 and provides it to the bundle sheath cells so efficiently, CO2 concentration within the bundle sheath cells is about 10 to 60 times as great as its concentration in the mesophyll cells of plants having only the C3 pathway.
- Photorespiration is negligible in C4 plants such as crabgrass because the concentration of CO2 in bundle sheath cells (where rubisco is present) is always high.
- The combined C3-C4 pathway involves the expenditure of 30 ATPs per hexose rather than the 18 ATPs used by the C3 pathway alone.
- The extra energy expense required to regenerate PEP from pyruvate is worthwhile at high light intensities because it ensures a high concentration of CO2 in the bundle sheath cells and allows them to carry on photosynthesis at a rapid rate.
- At lower light intensities and temperatures, C3 plants are favored.
- For example, winter rye, a C3 plant, grows lavishly in cool weather, while crabgrass cannot because it requires more energy to fix CO2.
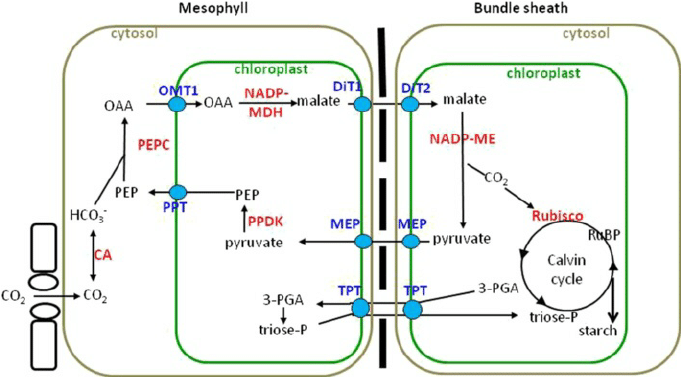
Fixation of CO2 by CAM plants at night:
- Plants living in dry, or xeric, conditions have a number of structural adaptations that enable them to survive.
- Many xeric plants have physiological adaptations as well, including a special carbon fixation pathway termed as the crassulacean acid metabolism (CAM) pathway.
- Unlike most plants, CAM plants open their stomata at night, permitting CO2 while minimizing water loss.
- They use the enzyme PEP carboxylase to fix CO2, forming oxaloacetate, which is converted to malate and stored in cell vacuoles.
- During the day, when stomata are closed and gas exchange cannot take place between the plant and the atmosphere, CO2 is removed from malate by a decarboxylation reaction.
- Now the CO2 is available within the leaf tissue to be fixed into sugar by the Calvin cycle (C3 pathway).
- The CAM pathway is very similar to the C4 pathway but with important differences.
- C4 plants initially fix CO2 into 4-carbon organic acids in mesophyll cells.
- The acids are later decarboxylated to produce CO2, which is fixed by the C3 path- way in the bundle sheath cells.
- In other words, the C4 and C3 pathways occur in several locations within the leaf of a C4 plant.
- In CAM plants the initial fixation of CO2 occurs at night.
- Decarboxylation of malate and subsequent production of sugar from CO2 by the normal C3 photosynthetic pathway occur during the day.
- In other words, the CAM and C3 pathways occur at various times within the same cell of a CAM plant.
- Although it does not promote rapid growth the way that the C4 pathway does, the CAM pathway is a very successful adaptation to xeric conditions.
- CAM plants can exchange gases for photosynthesis and reduce water loss significantly.
- Plants with CAM photosynthesis survive in deserts where neither C3 nor C4 plants can.