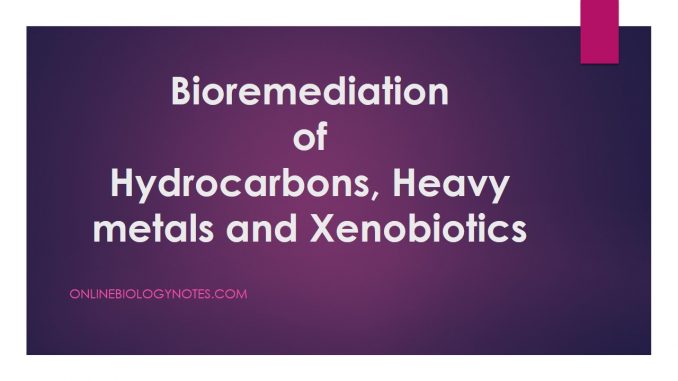
I. Bioremediation of Hydrocarbons
- Petroleum and its products are best examples of hydrocarbons and possess much economic importance.
- Oil constitutes of a variety of hydrocarbons, viz., xylanes, naphthalenes, octanes, camphor, etc. The excess amount of these cause pollution in the environment.
- If the growth conditions, e.g., temperature, pH and inorganic nutrients are as per need, micro-organisms can perform in toxic environment.
- Oil is less denser and insoluble in water. It floats on water surface and forms slicks.
- In storage tank microbial growth is not possible even if water and air are supplied.
- Various micro-organisms such as Pseudomonas, various Corynebacteria, Mycobacteria and some yeasts are capable of degrading petroleum.
- However, there are two methods for bioremediation of hydrocarbons/oil spills:
- By using mixture of bacteria
- By using genetically engineered microbial stains.
Bioremediation using mixture of bacteria:
- A large number of bacteria reside in interfaces of water and oil droplets.
- Each strain of bacteria uptakes a selective type of hydrocarbons, so, techniques have been developed to introduce mixture of bacteria not a single strain.
- Mixture of bacteria have been employed rapidly to control oil pollution in water or oil spills from ships.
- Bacteria residing in interface degrade oil at a very slow rate.
- The rate of degradation could be enhanced with human efforts.
- Artificially well-developed mixture of bacterial strain along with inorganic nutrients viz. phosphorus and nitrogen are pumped into the ground or put on to oil spill areas as needed for treatment. This accelerates the rate of bioremediation at target site.
Bioremediation using genetically engineered bacterial strains:
- Anand Mohan Chakrabarty, an India borne American scientist in 1979, found a strain of Pseudomonas putida that contained the XYL and NAH plasmid as well as a hybrid plasmid derived by recombinating parts of CAM and OCT (these are incompatible and cannot co-exist as distinct plasmids in the same bacterium).
- This strain had capability of metabolising hydrocarbons more efficiently than any other single plasmid thus it could grow rapidly on crude oil.
II. Bioremediation of industrial wastes:
- A large number of industrial units discharge different type of pollutants in the environment. For example, textile industry alone yields a large amount of pollutants to water resources such as enzymes, acids, alkali, alcohols, phenols, dyes, heavy metals, radionuclides, etc.
- Traces of zinc, cadmium, mercury, copper, chromium, lead are present in dyes.
- Bioremediation of toxic textile effluents can be performed by using either free living microbial system or immobilised microbial system and enzymes.
- Actinomycetes are more capable to bind metal ions in comparison to fungi and bacteria.
- Uptake mechanism of living and dead cells differ from bacteria to fungi.
- Due to these variation they have possible application in industries to treat industrial effluents.
- The living microbial cells collects metals intracellularly at a higher concentration, in contrast to dead cells that precipitate metals in and around cell walls by several metabolic processes.
- Aspergillus niger biomass consists up to 30% of chitin and glucan.
- Chitin phosphate and chitosan phosphate of fungi absorb greater amount of U than Cu, Cd, Mn, Co, Mg and Ca.
Bioremediation of heavy metals
- Micro-organisms like bacteria, algae, fungi, actinomycetes and higher plants gathers high concentration of heavy metals in their cells.
- Species of various microbial groups are discussed below:
Biomediation of heavy metals by Algae:
- Algal species are able to grow even in presence of heavy metals.
- The species of Chlorella, Anabaena inaequalis, Westiellopsis prolifica, Stigeoclonium tenue, Synechococcus sp. can grow in presence of heavy metals.
- This capability of these several species of Chlorella, Anabaena, marine algae are applied for the elimination of heavy metals from contaminated sites.
- Even if operational conditions restrict the practical application of these organisms.
- The naturally existing cells showed higher efficiency for Cd++ and Ni++ in comparison to laboratory cells.
Bioremediation of heavy metals by Fungi:
- Fungi also possess capacity to accumulate heavy metals in their cells and follow different mechanisms for removal of heavy metals from the solution.
- These mechanisms are explained below:
i. Metabolism-independent accumulation:
- The positively charged ions in the solution gets attracted to negatively charged ligands in cell materials.
- Biosorption of metal ion takes place on microbial cell surface.
- Some factors like make-up of biomass and other factors influence biosorption.
- In case of Rhizopus arrhizus, adsorption of some metals such as Li3+, Mn2+, Cu2+, Zn2+, Cd2+, Ba2+, Hg2+ and Pb2+ depends on the ionic radius. However, binding of Hg2+, Ag2+, Cd2+, A13+, Ni2+, Cu2+ and Pb2+ strongly depends on concentration of yeast cells.
ii. Metabolism-dependent accumulation:
- In this mechanism, heavy metal ions are carried into the fungal and yeast cells through cell membrane.
- In this metabolic processes ions are precipitated around the cells, and manufactured intracellularly as metal-binding proteins.
- Uptake of some metals ions such as Cu2+, Cd2+, Co2+, Ni2+, Zn2+ by fungi is energy-dependent.
- Similarly, certain external factors such as pH, anions, cations and organic materials, growth phase, etc affects intracellular uptake.
- in some fungi such as Aspergillus niger, Penicillium spinulosum and Trichoderma viride uptake of metals by growing batch culture reaches maximum during lag phase and early log phase.
iii. Extracellular precipitation and complexation:
- In this mechanism, fungi yields numerous extracellular products which are able to precipitate heavy metals.
- For example, many fungi and yeast manufacture and release high affinity Fe-binding compounds that chelate iron termed as siderophores.
- These siderophore chelates Fe3+ outside the cell wall and it is taken up into the cell.
- In Saccharomyces cerevisiae metals ion is eliminated out by their precipitation in their sulphides form, e.g., Cu2+ is precipitated as CuS.
Bioremediation of xenobiotics:
- In modern agriculture system, pesticides are being used excessively by modern society to enhance the quantity and quality of the food production across the world.
- It has become a common practice to use pesticide and it has become an integral part of modern agriculture system.
- These compounds present in pesticides are complex in structure and are made artificially, i.e., xenobiotics stays in environment and hesitate to undergo biological transformation.
- Micro-organisms can play a vital role in remediation of xenobiotics as they have potential to degrade, and maintain stable state concentrations of chemicals in the environment.
- An oxidative cycle is required to eliminate the potential toxicity of the pesticide yielding inorganic components by its complete degradation.
Objectives for biodegradation of xenobiotics:
- To understand the mechanism of micro-organisms of how they perform in presence of such complex compounds.
- To develop bioremediation methods for eliminating or detoxifying high concentration of hazardous pesticide residues.
- The characters of pesticide degradation using micro-organisms are present on plasmids and transposons, and are classified in clusters on chromosome.
- Understanding of the characters provides important information inorder to develop degradative pathways and makes the task of gene manipulation easier to make the genetically engineered microbes capable of degrading such complex compounds as pollutants.
Microbial degradation of xenobiotics:
- Biodegradation of pesticides takes place by aerobic micro-organisms under aerobic conditions.
- Pesticides are regarded as of wide varieties of chemicals, e.g., chlorophenoxyalkyl caboxylic acid, substituted ureas, nitrophenols, triazines, phenyl carbamates, organochlorines, organophosphates and many more
- The most commonly used insecticides in agriculture practices are organophosphates (e.g., diazion, methyl parathion and parathion).
- Biodegradation through hydrolysis by Pseudomonas diminuta and Flavobacterium are regarded as the most important steps in the detoxification of organophosphorus compounds.
- Organomercurials (e.g., Semesan, Panodrench, Panogen) have been applied in agriculture since the origin of fungicides.
- Various species of Aspergillus, Penicillium and Trichoderma have been isolated from Semesan treated soil.
- They showed ability to grow over 100 ppm of fungicide in vitro. The major fungicides employed in agriculture are water soluble derivatives such as Ziram, Ferbam, Thiram and can be degraded by micro-organisms.
- Pentachlorophenol (PCP) is a wide spectrum multipurpose biocide which can be used as fungicide, insecticide, herbicide, algicide, disinfectant and antifouling agent.
- Bioreactors using alginate immobilised and polyurethane foam immobilised PCP degrading Flavobacterium cells can be used to remove PCP from contaminated water.
- To decrease the toxicity of PCP, absorption of PCP by polyurethane immobilised matrix plays a vital role.